MAGIC observations of high-peaked BL Lacertae objects
Volltext
Abbildung
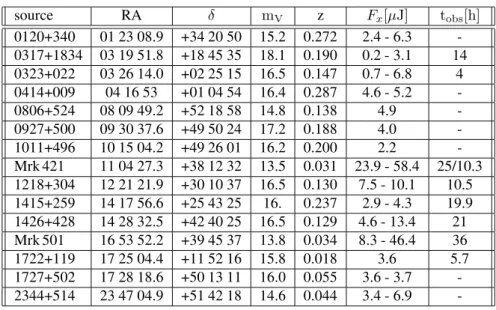
ÄHNLICHE DOKUMENTE
Bartko et al., Search for Gamma Rays from the Galactic Center with the MAGIC telescope in proceedings of 29th International Cosmic Ray Conference (2005) [astro-ph/0508244].
Emphasis is put on the stability of the flux determination during periods with different telescope performances and on describing new analysis methods used to extract signals in the
Those energetic systems are believed to be as well γ-ray emitters, not only by interaction between the two stars [15][7] but also, several models of millisecond pulsars [4]
For the spectrum calculation the data taken between December 2004 and April 2005 (samples I+III only) have been divided into a high and a low flux state (highest 4 and lowest 7
In the MAGIC telescope the measure of the absolute time is provided by a high-precision atomic clock (Rubclock), formed by a Rubidium standard frequency oscillator in conjunction with
The MAGIC telescope is a single mirror instrument designed to detect the Cherenkov light produced by the electromagnetic cascades initiated by incoming high energy
a) It has the worldwide largest mirror dish. b) It is the first time that a lightweight mirror dish has been constructed from carbon fiber reinforced plastics (CFRP). c) First use
The intrinsic (corrected for the EBL absorption) energy spectrum of Mkn 180 is shown in Fig. 2 together with simultaneous and historical data. The VHE γ -ray spectrum is within