Interference of transcription on H-NS mediated repression in Escherichia coli
87
0
0
Volltext
(2)
(3)
(4)
(5)
(6)
(7)
(8)
(9)
(10)
(11)
(12)
(13)
(14)
(15)
(16)
(17)
(18)
(19)
(20)
(21)
(22)
(23)
(24)
(25)
(26)
(27)
(28)
(29)
(30)
(31)
Abbildung
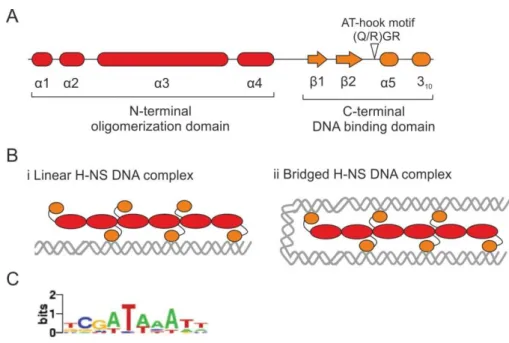



+7
ÄHNLICHE DOKUMENTE
Still ring shaped structures are formed, but these structures appear similar to the illumina- tion of the other materials at high laser intensities, where
Det er praktisk at få vist 9 fotos, når du skal vælge fotos, der skal udskrives ved brug af Print Range of Photos (Udskriv en række fotos) eller angive indstillinger for View
(VIL DU BENYTTE DETTE PAPIR?) vises på LCD-displayet, skal du trykke på knappen Menu for at vælge mediet og derefter trykke på knappen Enter for at indstille mediet..
im relativ unpolaren Wasser/Acetonitril-Gemisch → im relativ polaren Wasser/Acetonitril-