Synthesis and biological evaluation of a compound collection inspired by withanolides
Volltext
Abbildung
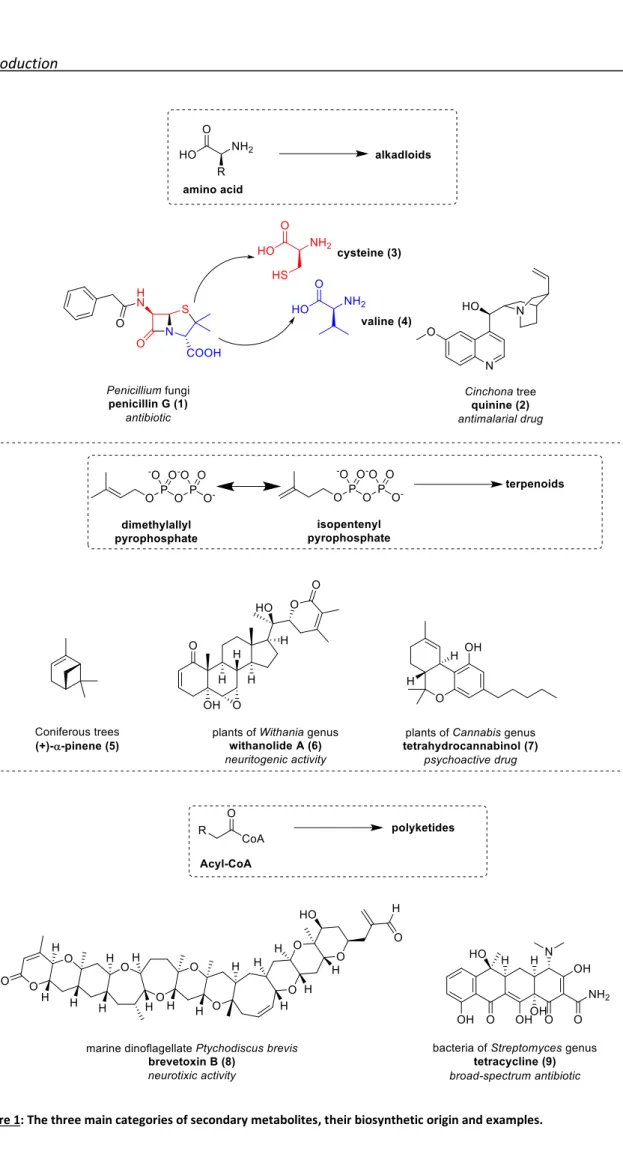
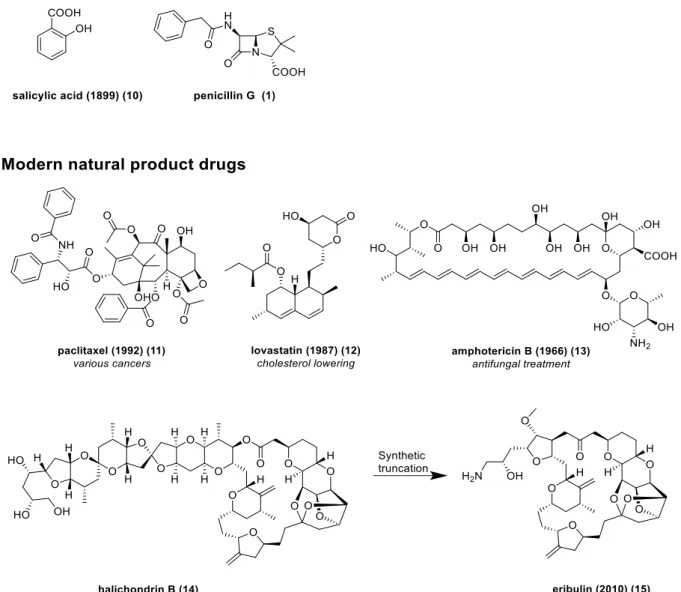
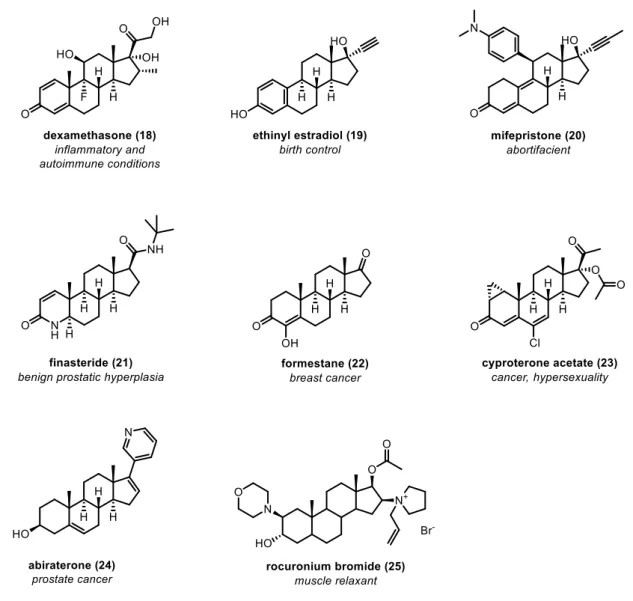

ÄHNLICHE DOKUMENTE
cabralealac- tone 3-acetate-24-methyl ether, was isolated from the aqueous methanolic extract of For- sythia koreana fruits, along with eight known compounds:
(B) Analysis of cell death type (apoptosis and necrosis) in MCF-7 cells treated with the test extract or compounds, at their respec- tive IC 50 value, or with TRAIL (80 ng/ml),
The organic layer was separated, dried over anhydrous MgSO 4 , filtered, and concentrated under reduced pressure.. The reaction mix- ture was evaporated in vacuo, and the residue
The organic phase was separated, washed with a saturated aqueous NH 4 Cl solution (10 mL), dried over an- hydrous MgSO 4 and concentrated under reduced pressure.. The residue
Determination of the geographic origin of poultry meat and dried beef (with respect to both raw meat origin and place of processing) using element and oxygen isotope
Determination of raw Determination of raw meat meat origin origin and place and place of processing of processing of of dried dried beef beef is is possible?. possible in in
It was possible to distinguish between samples of Brazilian raw meat origin, when processed in different countries, but samples from other raw meat origins, processed in
59 dried beef samples from Australia, Austria, Canada, France, Switzerland and USA (raw meat origin and place of processing identical except Austrian (Brazilian raw meat) and