Characterization of an olfactory receptor mediating aversive behaviour to a death-associated odour
Volltext
Abbildung
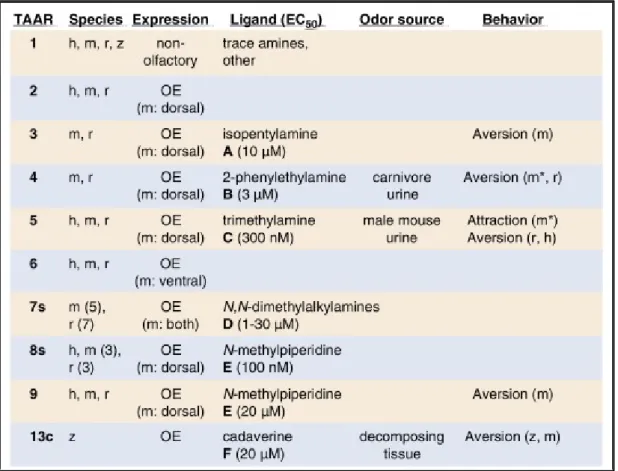
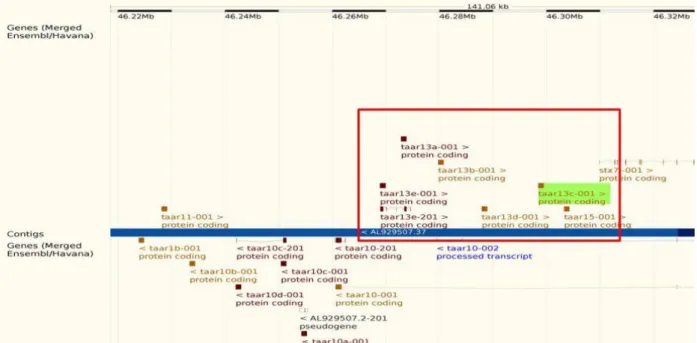
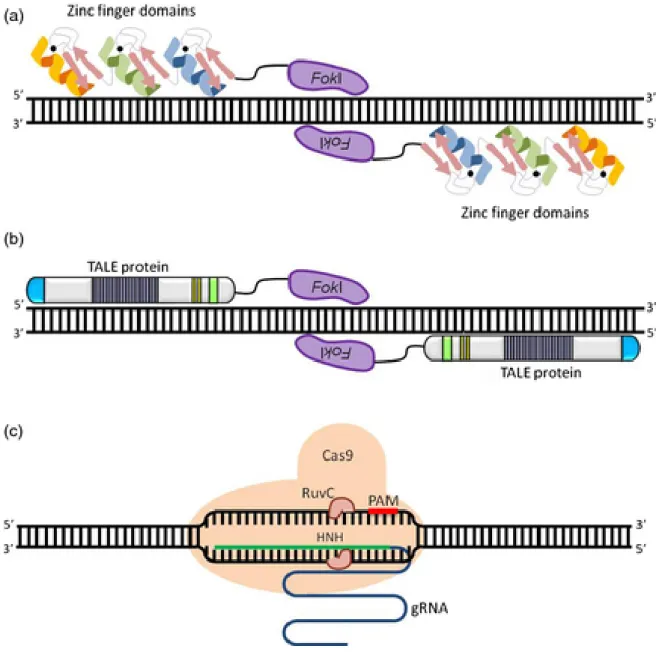
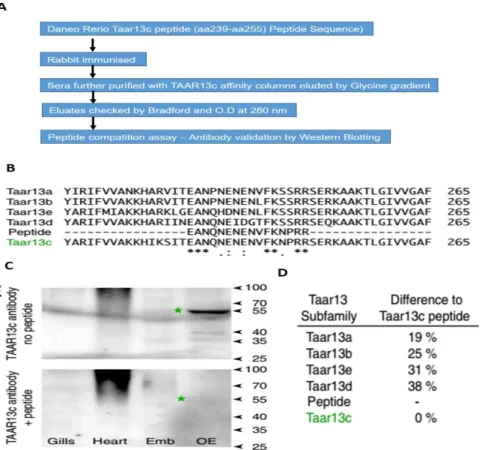
ÄHNLICHE DOKUMENTE
There are various mechanisms of interest speculated to be involved in the observed staining of ORNs namely; via diffusion through open cAMP/CNG cation channels, and
No odorant-induced responses could be obtained from isolated ORNs recorded in the on-cell mode, while recordings in the slice (on-cell and whole-cell) as well as previously
TABLE 1 Average and maximum C stocks in living and dead volumes for forest registered as managed and unmanaged in Germany, based on plot data from the national forest
Correlation analysis demonstrated that MC population activity patterns evoked by Arg and His (pure odors) were initially dissimilar (R = –0.25 at 256 ms) and remained
predictors were centered according to Kraemer and Blasey (2004), and interaction terms were.. residuals-centered according to Little et al. Number of stressful life events were
After the expression of OR and V1R genes was verified in the olfactory tissue, a single cell RT-PCR was conducted to validate whether single or multiple chemoreceptors are
Membranes prepared from insect cells expressed the 5-HT 7(a) receptor wild type and different C-terminal cysteine mutants were incubated with [ 35 S]-GTPγS in the presence or
Besides the contribution of mast cells to allergic inflammation [132], they also seem to be involved in non-allergic inflammatory diseases of the gut, since in the intestine of