A Theoretical Framework of Haptic Processing in Automotive User Interfaces and Its Implications on Design and Engineering
Volltext
Abbildung

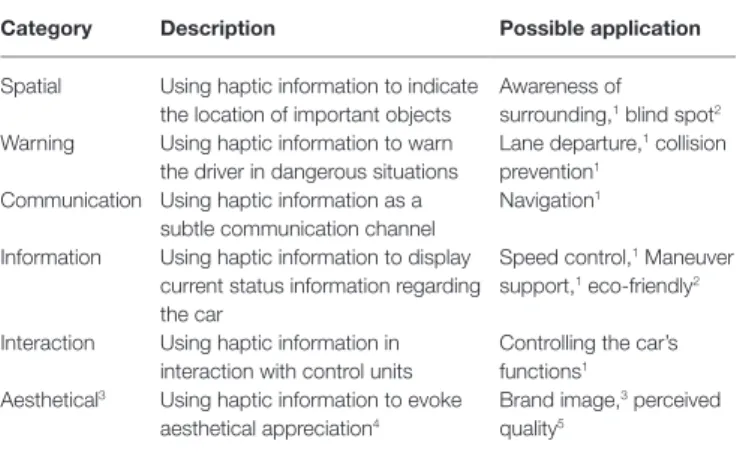

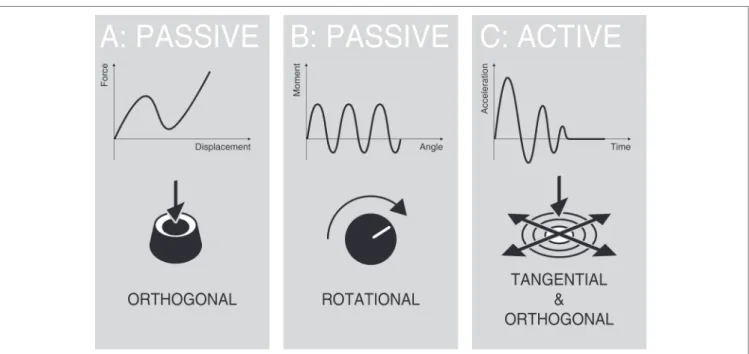
ÄHNLICHE DOKUMENTE
describes an organizational scheme for interaction design patterns to support the process of implementing a complete pattern language covering all the different levels of the solution
In this work our lumbar puncture simulator prototype presented in [FHH07, FHD + 08] has been extended by a multiproxy haptic rendering technique, new visualization techniques and
Concur Task Trees that have been identified in the analysis phase of the design process provide the basic user interface structure and are evolved step by step within the
Mit geeigneten Architekturen für einen flexiblen Software Download von Fahrzeugfunktionen in der Entwicklung, der Produktion und der Wartung beschäftigt sich der Beitrag
Abstract: This paper presents a simple method for the initial design and validation of graphical user interfaces by group exercises based on brainstorming and story- boarding with
In chapter one, after reviewing the relevant theoretical and empirical literature, I develop a framework using varieties of capitalism [VOC], state size, and resource geography
This architecture enables systematic exploration of motor imagery BCI enhanced with both haptic feedback and live visual dance feedback (specifically comparing four conditions;
To measure the effect of the presented modalities on users’ presence we conducted a study with 80 participants.. Participants were recruited through postings in the university