Tumorantigen-Specific CD40B Cells: Combining Enhanced Antigen-Presentation and Antibody-Secretion for Tumor Targeting
Volltext
Abbildung
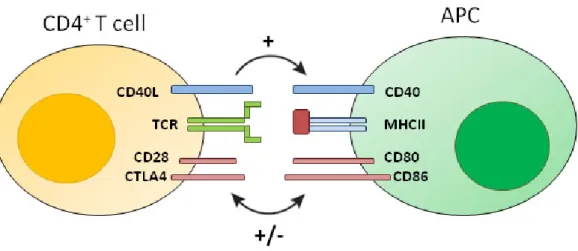
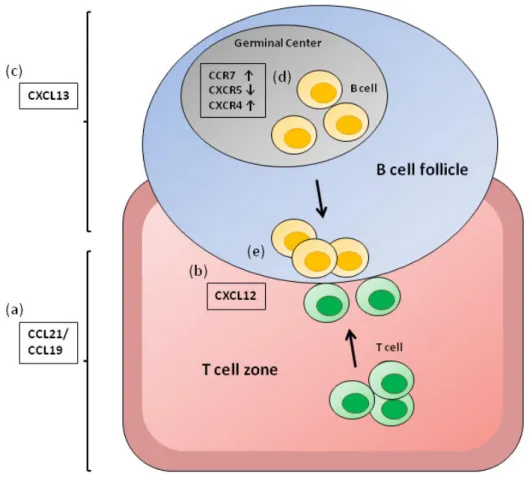
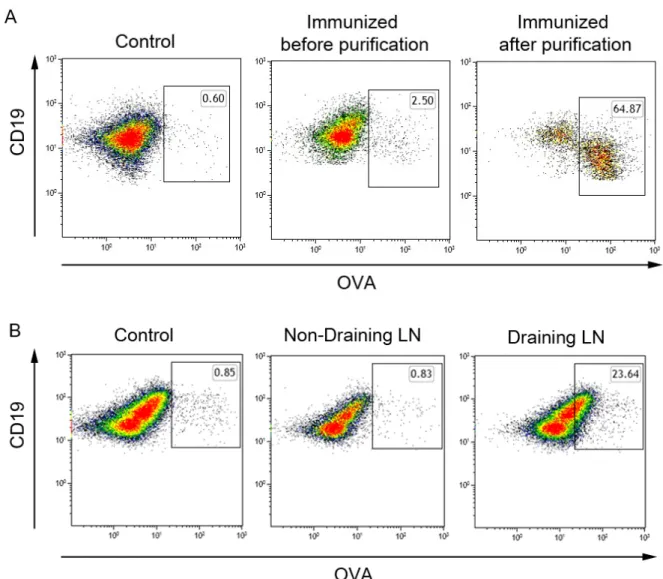
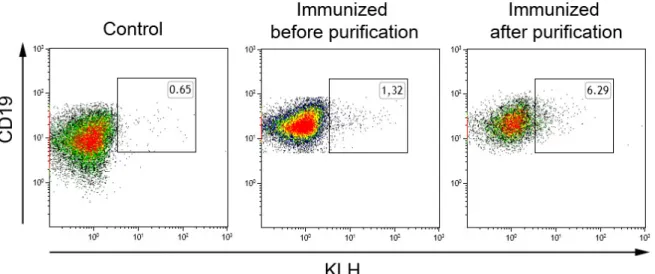
ÄHNLICHE DOKUMENTE
RESULTS: We show for the first time that EC present a quantitatively different peptide repertoire with abundance of certain peptides, compared with leukocytes. The abundance of
Among the CD1 family, CD1e is the only soluble protein, thus supporting a chaperone-like rather than an antigen-presenting function. Furthermore, CD1e is never
and the enormous sensitivity of chromium release Measuring activation of hybridomas started at 10 27 – assays are not ideal prerequisites for monitoring the 10 28 M concentrations
We did not detect secretion of suppressive cytokines (such as IL-4, IL- 10 or TGF-~) from CDS' T cells primed by LSEC that could ex- plain the Joss of specific T-cell
Minami et al. not only found BAG6 associated with chemically induced DRiPs, they
To analyze the influence of BAG6 on MHC class I surface expression, the murine fibroblast cell line B8-D b (H- 2 d + H-2D b ) and HeLa cells were subjected to BAG6
To exclude that the reduced number of virus-specific T cells in Bis VIII–treated mice at day 7 after viral infection was due to inhibition of T cell activation and reduced expansion,
and our phase I trial in cervical cancer patients [25], has demonstrated the efficacy of autologous tumor lysate pulsed DCs (TLDCs), as a next step after optimizing the rhSPAG9