poly(dimethylsiloxanes) and other polymers: Development and optimization of a polymer HPLC method
Volltext
Abbildung
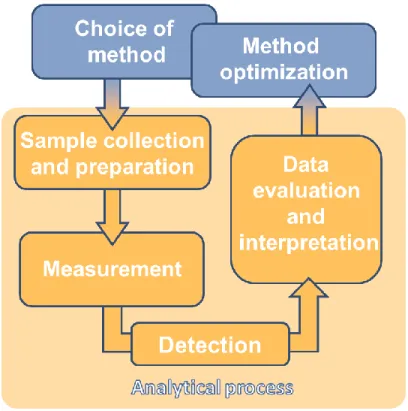
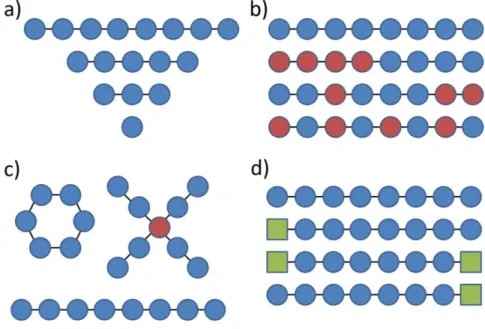

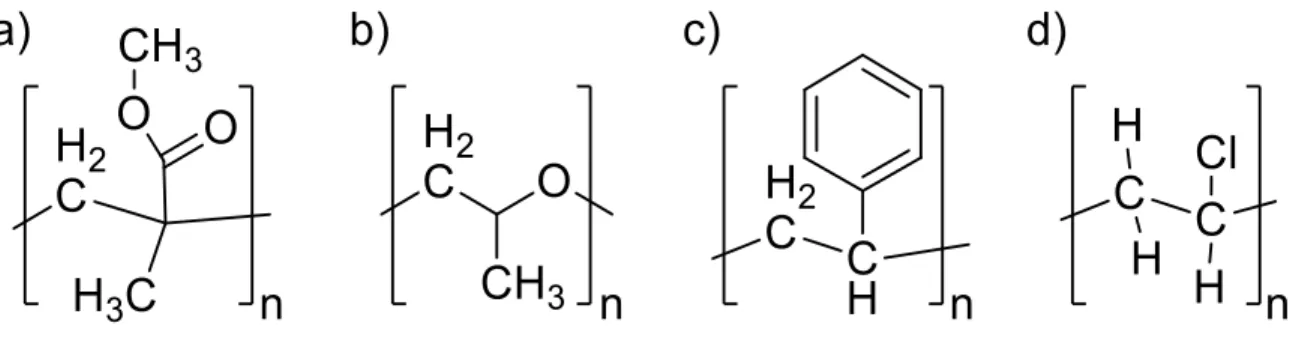
ÄHNLICHE DOKUMENTE
DOC dissolved organic carbon (gelöster organischer Kohlenstoff) DON dissolved organic nitrogen (gelöster organischer Stickstoff) DOM dissolved organic matter
scholaris, as well as the fractions of the ethanol- ic extract of the leaves (viz., n-hexane, chloroform, ethyl acetate, n-butanol, and remaining water), were tested in vivo
Figure 45: Newtonian and non-Newtonian field recovery factor for different polymer concentration The discrepancy in recovery factor values shown in Figure 45 emphasis
Since in application in CLD laminates damping is provided via shear defor- mation of the adhesive damping layer when the structure is bent, a DMA setup in shear mode was used
To sum up, the suggested process control for polymeric flocculant addition is based on two parameters: the Residual Flocculant Parameter (RFP), which controls possible toxic effects,
The analytical model which uses the fast queuing simulation trace results is able to produce final results of the impact of transport flow control and congestion control on
Furthermore, in gradient elution separations of a simple pharmaceutical drug mixture, for example, a peak width decrease of ~15% and a resolution increase of up to 33% were
Figure 6.3: (A) Diffraction micrograph collected at 33.3 cm camera length, (B) Structure factor, (C) the corresponding radial distribution function for liquid water. Please