Cell death and transcriptional signalling mediated by the coiled-coil domain of the barley resistance protein MLA
Volltext
Abbildung
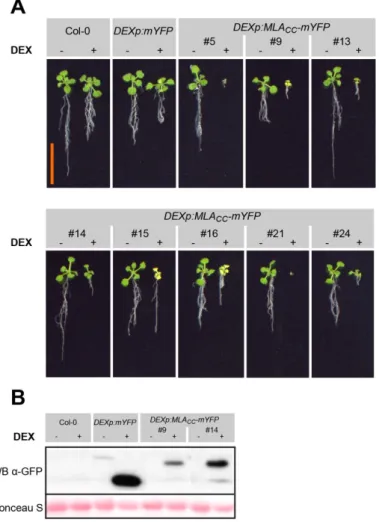
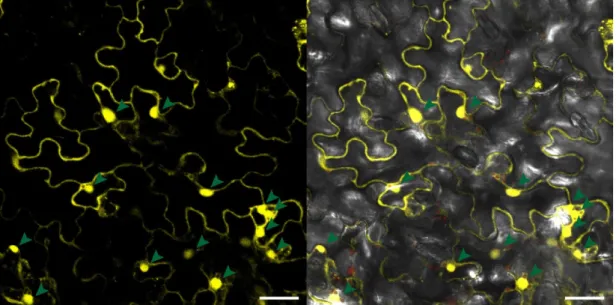
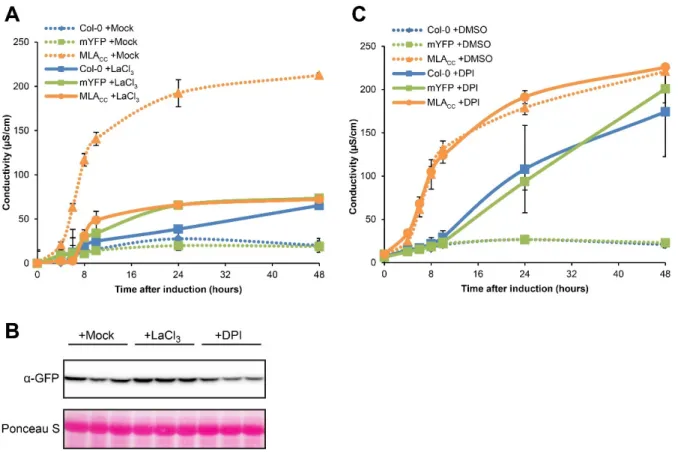
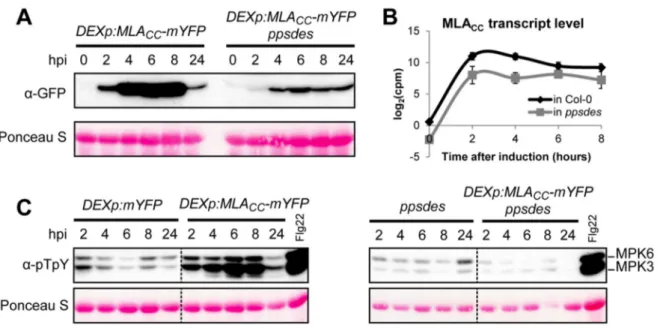
ÄHNLICHE DOKUMENTE
PD treated patients have shown decreased levels of naïve T cells, Tregs, dendritic cells, Th1, Th2, and Th17 cells, as well as increased counts of effector T, memory T, Th1,
In contrast to inflamma- tory cells, accelerated cell death is often observed in epithelial cells, a mechanism, which amplifies or at least maintains allergic inflammation..
Our observations strongly suggest that HAMLET stimu- lates a regression of epithelial structures and an apoptotic cell death of secretory epithelial cells in the lactating mammary
An increasing number of examples shows that bacterial pathogens utilize their secreted proteins to dampen host innate immune responses by interfering with two
Overexpression of Gli1 and Gli2 as the primary mediators of hedgehog signaling has no effect on the expression of the surface death receptor proteins Fas, TNFR
We first analyzed whether the expression of total H2AX was altered, and did indeed find that this molecule was reduced in CD8 + CD28 ) T cells when compared to CD8 + CD28 + T cells.
As mitochondria play a crucial role in the regulation and induction of apoptosis because of their ability to release several pro-apoptotic proteins, the Bcl-2 family of proteins,
Our findings strongly suggest that increased leakiness of the nuclear barrier affects nucleocytoplasmic transport, alters the localization of proteins across the