Generation of an oncolytic adenovirus vector combining three cancer targeting strategies and characterization of a new preclinical model for breast cancer virotherapy
Volltext
Abbildung

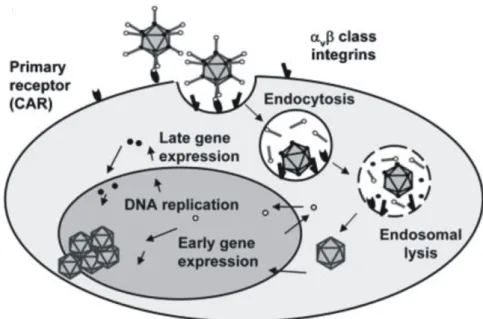
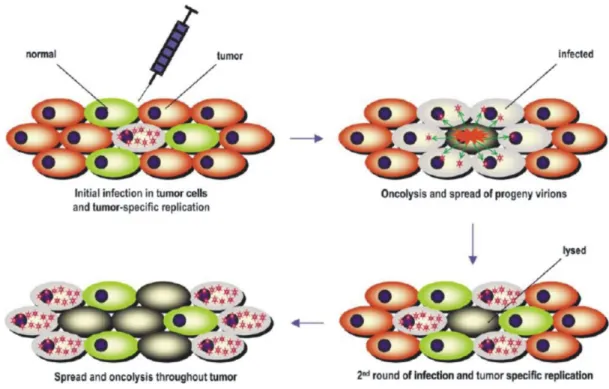
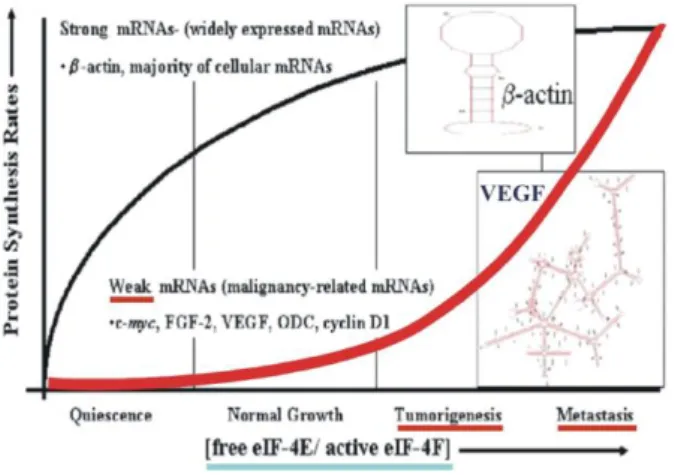
ÄHNLICHE DOKUMENTE
Direct comparison of various cell lines and measles virus strains has only been performed by (Scott and Choppin, 1982), so far, who documented the highest yields using Vero
Because CA15-3 was more frequently obtained for the more recently conducted trials, we found that the median follow-up and percentage of patients with recurrence was greater
Expression of E545K and H1047R mutant p110α in the immortalized but non-transformed mammary epithelial cell line MCF10A increased the PI3K kinase activity, allowed
Bei Patientinnen mit einem deutlich erhöhten Risiko für die Entstehung einer Os- teoporose oder bei bekannter Osteoporose sollte eine entspre- chende Medikation entsprechend
Table 5 Odds ratios (ORs) and 95% confidence intervals (CIs) for the associations between age, parity, body mass index (BMI), family history, and levels of the Nottingham
(ii) the PROGNOSTIC collection ( N ¼ 826), including node negative patients with early breast cancers not treated with any systemic therapy until relapse; (iii) the TAM
The aims of this article were therefore (a) to describe the process behind a free, not-for-profit commu- nity-based therapeutic exercise and education programme (TEEP) for BCS in
(2) changes in cell fate by epithelial-mesenchymal transition (EMT) including a transforming growth factor beta (TGF β )-mediated switch of E-cadherin to N-cadherin expression