The creation of self-assembled organic nanotubes (S-ONTs) represents a field of research that has attracted much attention
Volltext
Abbildung
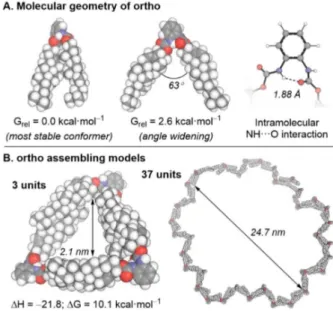
ÄHNLICHE DOKUMENTE
The paper reviews and evaluates eight research methods or approaches that include some form of synthesis (literature review, systematic review, meta-analysis, meta-ethnography,
The META-NET Language White Paper Series “Eu- rope’s Languages in the Digital Age” (Rehm and Uszko- reit, 2012) describes the current state of LT support for 30 European
In light of the asymmetry in market size between the two economies, MERCOSUR and the United States, the original conjecture of this study was that exports from the United States
The steady state and dynamic photophysical characteristics of -cyanostilbene derivatives have been investigated in solution and in polyvinylchloride (PVC) film at 300 K as well as in
On 19 May 2015, Geneva will celebrate the two-hundredth anniversary of its accession to the Swiss Confederation. This occasion provides an opportunity to reflect on how the past
For the aforementioned reasons, the present thesis aims to further contribute to the analysis of her fiction as specifically Gothic by exploring how du Maurier
The META-NORD reports aim at raising aware- ness for language technology support and the ben- efits of sharing and exchanging resources by de- picting the importance of
After brief introductions to corpus linguistics and the concept of meta-argument, I describe three pilot-studies into the use of the terms Straw man, Ad hominem, and Slippery