Spectroscopic studies on AGNs and High angular resolution in the NIR: The construction of an imaging beam combiner for the LBT
Volltext
Abbildung


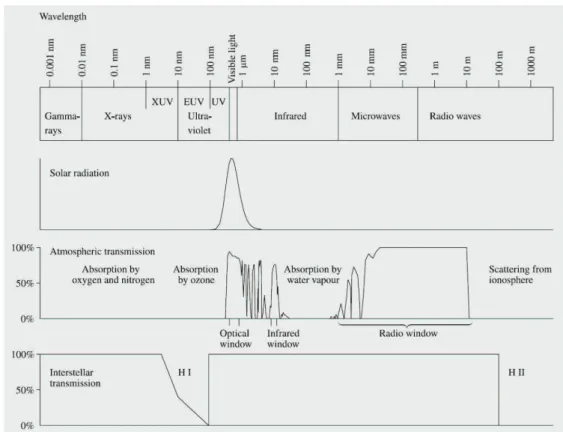
![Figure 2.1: Microphotometer tracings of the emission lines λ 4860 Hβ, 4959 and 5007 [O iii ] in the nebula NGC 1068 from Carl Seyfert,1943, (Seyfert 1943).](https://thumb-eu.123doks.com/thumbv2/1library_info/3697464.1505859/26.892.136.741.145.496/figure-microphotometer-tracings-emission-lines-nebula-seyfert-seyfert.webp)
ÄHNLICHE DOKUMENTE
a) Reduction in the number of dental visits and treatment provided by the private sector, in an effort to reduce pri- vate spending leading to a sharp decline in the income of
3 Franklin Allen, Historical Background, (1999), ‘How Financial System Differ and How They Develop?’, Wharton School, working paper pp1.. 2) The provision of a mechanism for pooling
They also published a few literary and cultural writings from the Global South, mainly Latin American authors, like Gabriel García Marquez (Feltrinelli published the first
Photography and the artistic construction of the contemporary” (2022), the guest editor is interested in exploring the contemporary relations between photography and time, through
The results suggest that higher taxes indeed increase the share of low income earners' share in total gross income and reduce the respective share of top income earners..
In, for instance, the third Venician edition of the Babylonian Talmud (1550), tractates of Derekh 'Eretz were admitted. See also the survey of the rendering of the Derekh
The individual construction phases of the proposal for the construction of the cased step pyramids using steep ramps tangentially attached to the sides and conversions
Hathor is linked with desert animals much more than any other ancient Egyptian deity, probably because her conceptualisation as the star Sirius (invisible for part of the year)