Effect of different mutations in the ATM gene on the cellular response to ionizing radiation
Volltext
Abbildung
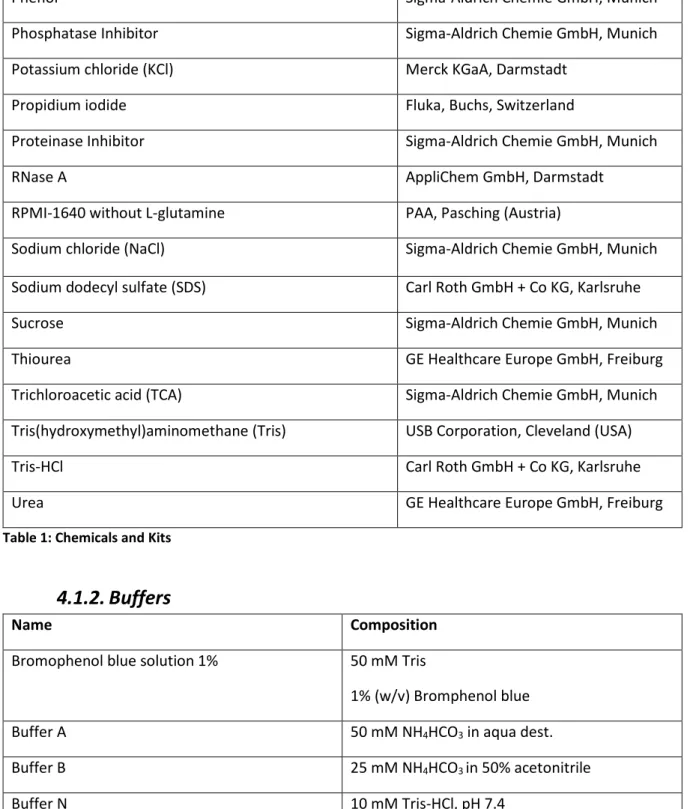
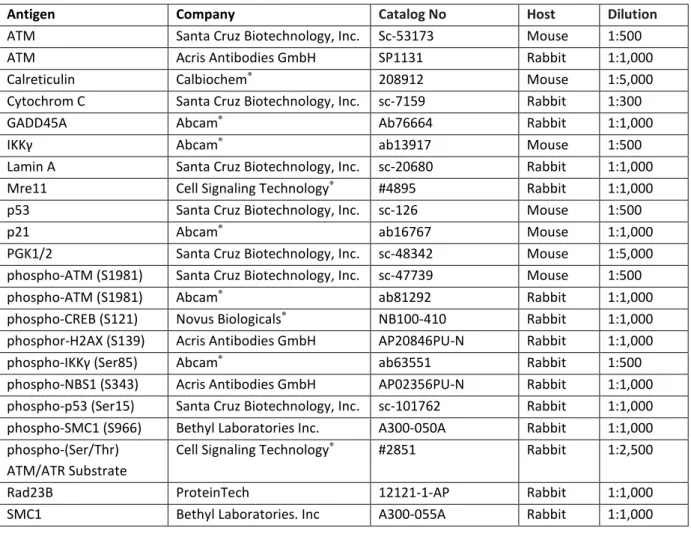
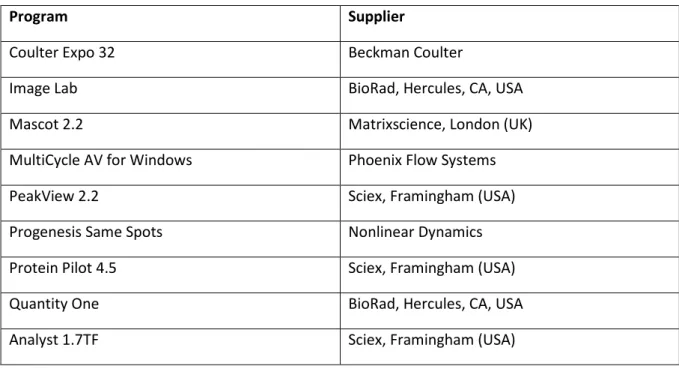
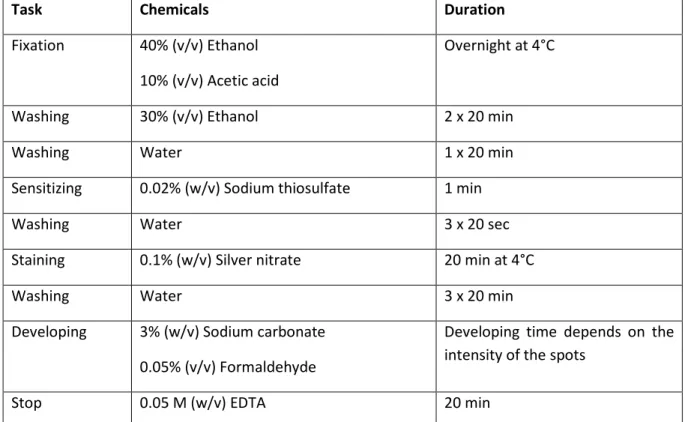
ÄHNLICHE DOKUMENTE
Additionally, within the irradiated samples four different characteristic representatives (without ectoine, 0.2 Gy and 14.07 Gy respectively/ectoine solution, 0.26 Gy and 15.83
Currents, number of primary electrons are measured, the calculation of irradiation doses is based on Hahn et.. All samples are analyzed by
Our real-time PCR experiments confirmed the results of the microarray analysis that OSTL is expressed at lower levels in ALL than in myeloid leukemia, especially CML.. The ALL
To test for a potential role of the demethylase in the DNA damage response, cell cycle analysis experiments and recruitment kinetics of damage response proteins
In my thesis I have proposed and developed a statistical approach that allows study- ing molecular mechanisms of temporal gene expression responses from a time-course
The frequency of detected mutations in the known genes associated with hereditary pancreatic cancer is difficult because patients with known deleterious variants in the
Whereas the Notch2 +/HCS mutation favors pro-osteoclastogenic gene expression, triggering a high bone turnover pathology, the Wnt1 G177C/G177C mutation specifically affects
In the present study I analyzed the signature of miR-16, miR-30b and miR-93 in exosomes derived from plasma of BC patients and compared it with that in DCIS patients and healthy