oogenesis and early embryogenesis in selected nematode species
Volltext
Abbildung
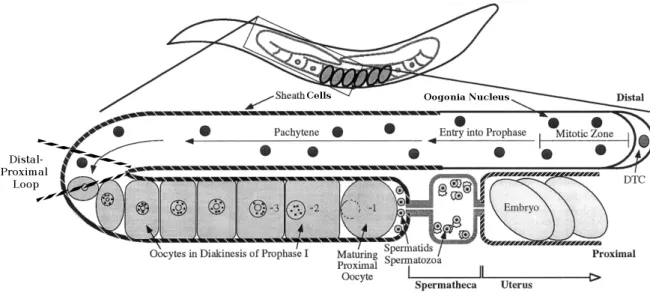
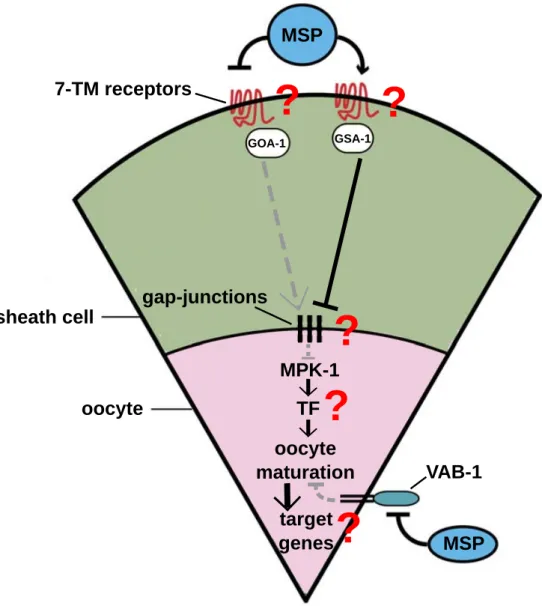
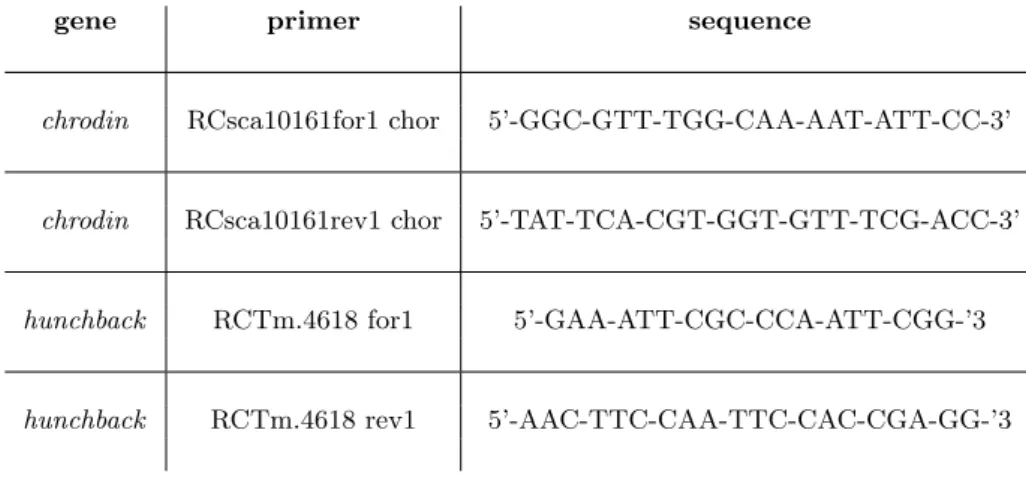
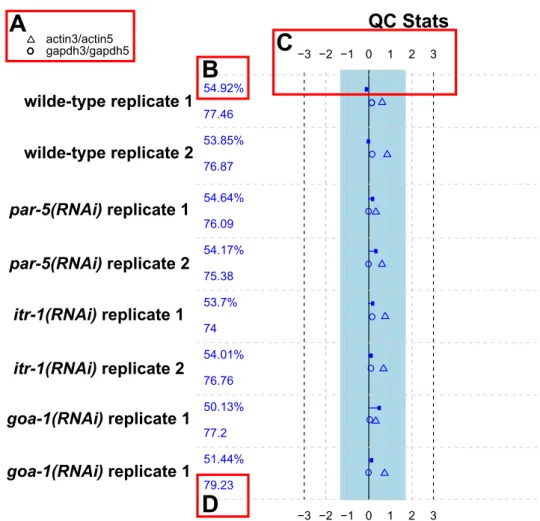
ÄHNLICHE DOKUMENTE
The Garage Journal: Studies in Art, Museums & Culture is launching a grant program to support independent and early career researchers engaged in writing on the arts and
Accordingly, the aim of the present dissertation was to integrate the hormonal and psychological approaches of training monitoring during the course of the preparatory as well as
The EDIT Specimen Explorer, the second component examined, helps find taxonomically relevant specimen and observation data by searching the GBIF (Global Biodiversity
Part I of this paper adopts the frame- work of policy coherence for sustainable development (PCSD) to examine how countries are addressing, first, the integrated nature of the
Co-crystal structure of the complex of Bnip-FH2 with muscle actin suggests that the FH2 domain can stabilize actin dimers or trimers, as a likely mechanism for polymerization
FRAP experiments have shown that Slam is highly mobile at the onset of cellularisation but is quite stably associated with the membrane during cycle 13 and cycle
Drawing on existing literature, an early-career researcher workshop, and our own experience as junior research group leaders, we discuss specific challenges and respective
Conclusion: As our field develops it is crucial for the ELSI community to continue looking forward to identify how emerging digital solutions can be used to facilitate the