Identification and characterization of a genomic region involved in Drosophila gastrulation
Volltext
Abbildung



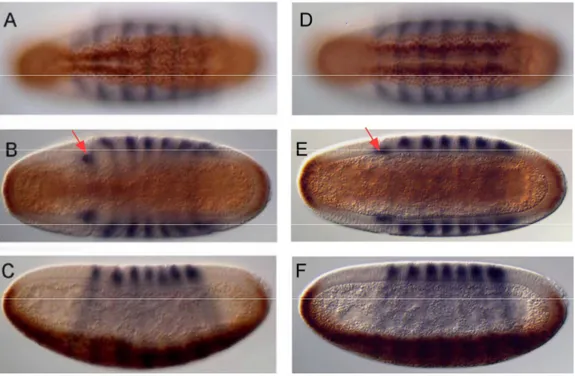
ÄHNLICHE DOKUMENTE
In conclusion, this project allowed to identify several candidate genes using microdissected tissues to not only provide global information on gene expression
Accumulating evidence, however, suggests that the mechanosensory cells have evolved from a common ancestral cell [Fritsch & Beisel 2004]: The mechanosensory cells of
Subsequently, the parallel reporter gene assays were used to quantify the regulatory activity of chromatin elements with predictive features of regulatory function at SNPs within
The Swiss Federal Electricity Commis- sion (ElCom) and the Swiss Federal Office of Energy (SFOE) have decided to conduct a joint consul- tation concerning the question of a
Correlation analysis of an allele and the flowering time phenotype Allele sequences of the progeny of the mapping population GF.GA-47-42 x ‘Villard Blanc’.. were compared against
MDD patients carrying the short (s) allele of the 5 HTTLPR showed increased brain serotonin turnover compared to those carrying the long (l) allele at this locus (Barton et al.,
After identifying the genes that showed a difference in expression between skin samples of the different color morphs of PS, we used qRT-PCR to confirm and expand upon the results
Differential Expression of SSH Candidates in Developing Swords and Gonopodia and Regenerating Caudal Fins Our gene expression analyses of seven clones from