The Diphosphorus Complex [Cp Cr (CO) ( -P )] as a Building Block in the Coordination Chemistry of Silver
Volltext
Abbildung
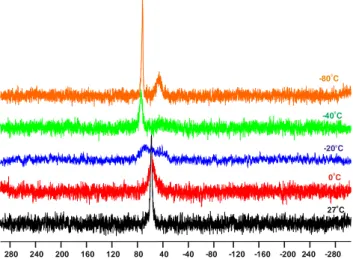
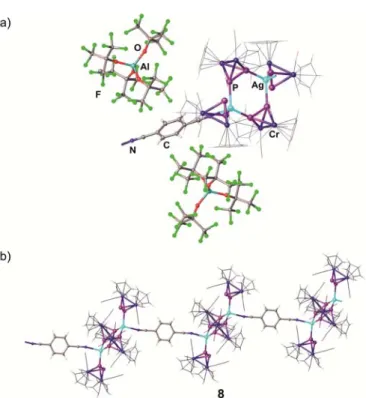
ÄHNLICHE DOKUMENTE
With the aim to prepare a structurally designed catalyst, we investigated the complexation of a ligand with a phenyl substituted pyrazine to see if the
The fact that these catalysts showed high selectivity in the hydrogenation of many different substrates such as 1.21, 1.22 and 1.23 highlights the importance of
In conclusion, the comparison of intermolecular in- teractions existing in the crystals of a series of dif- ferent compounds indicates that the dominant stability of
6 the depen- dence of the crystal-field strength parameter N ν ( B 2q ) on the excitation energy is shown for Eu(III) com- plexed to HS (GoHy 573 FA, bold lines) and compared to
To study the local structure distortion of the CrP 10− 4 cluster in the InP:Cr 2+ system, it is neces- sary to know the values of the crystal field parameters and the Racah
The strong decrease of the emission anisotropy in the SE band located around 330 – 350 nm observed by different authors for CDMA may be a result of the overlapping with the weak
The ligand N,N'-dimethylurea (DMU) is used to propagate the octahedral coordination geom- etry of [Co(DMU) 6 ] 2+ into 1D and 2D assemblies via a combination of coordinative bonds
A further distinction of (i) lake water, and (ii) suspended solids in the water body; (iii) pore water and (iv) sediment in the bottom layer allows a clear indication of